Virtual agriculture: development and transfer of agricultural technologies in the 21st century
- GREENBAR
- Dec 17, 2023
- 26 min read
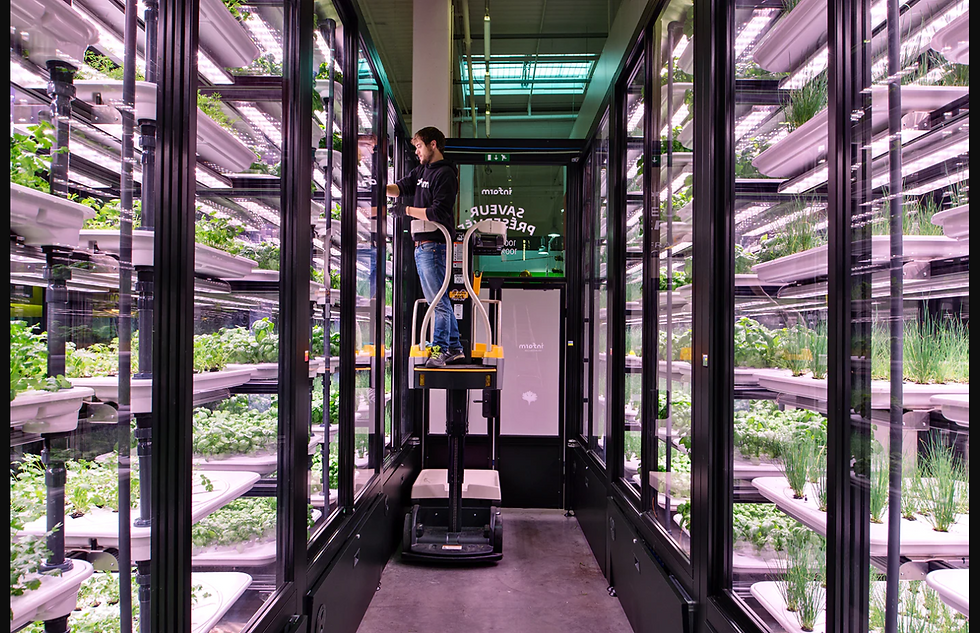
PROGRESS ON IMPROVING AGRICULTURAL NITROGEN USE EFFICIENCY: UK-CHINA VIRTUAL JOINT CENTERS ON NITROGEN AGRONOM
Zhaohai BAI2 ,
Zejiang CAI3 ,
Weidong CAO4 ,
Alison CARSWELL1 ,
Nicholas COWAN5 ,
Zhenling CUI6 ,
David CHADWICK7 ,
Bridget EMMETT8 ,
Keith GOULDING9 ,
Rui JIANG10 ,
Davey JONES7 ,
Xiaotang JU6 ,
Hongbin LIU11 ,
Yuelai LU12 ,
Lin MA2 ,
David POWLSON9 ,
Robert M. REES13 ,
Ute SKIBA5 ,
Pete SMITH14 ,
Roger SYLVESTER-BRADLEY15 ,
John WILLIAMS15 ,
Lianhai WU1 ,
Minggang XU11 ,
Wen XU6 ,
Fusuo ZHANG6 ,
Junling ZHANG6 ,
Jianbin ZHOU10 ,
Xuejun LIU6
Author information +
History +
Highlights
● Virtual joint centers on N agronomy were established between UK and China.
● Key themes were improving NUE for fertilizers, utilizing livestock manures, and soil health.
● Improved management practices and technologies were identified and assessed.
● Fertilizer emissions and improved manure management are key targets for mitigation.
Abstract
Two virtual joint centers for nitrogen agronomy were established between the UK and China to facilitate collaborative research aimed at improving nitrogen use efficiency (NUE) in agricultural production systems and reducing losses of reactive N to the environment. Major focus areas were improving fertilizer NUE, use of livestock manures, soil health, and policy development and knowledge exchange. Improvements to fertilizer NUE included attention to application rate in the context of yield potential and economic considerations and the potential of improved practices including enhanced efficiency fertilizers, plastic film mulching and cropping design. Improved utilization of livestock manures requires knowledge of the available nutrient content, appropriate manure processing technologies and integrated nutrient management practices. Soil carbon, acidification and biodiversity were considered as important aspects of soil health. Both centers identified a range of potential actions that could be taken to improve N management, and the research conducted has highlighted the importance of developing a systems-level approach to assessing improvement in the overall efficiency of N management and avoiding unintended secondary effects from individual interventions. Within this context, the management of fertilizer emissions and livestock manure at the farm and regional scales appear to be particularly important targets for mitigation.
Graphical abstract
Keywords
CINAg / N-CIRCLE / nitrogen use efficiency / reactive nitrogen / sustainable production
Cite this article
Download citation ▾
Tom MISSELBROOK, Zhaohai BAI, Zejiang CAI, Weidong CAO, Alison CARSWELL, Nicholas COWAN, Zhenling CUI, David CHADWICK, Bridget EMMETT, Keith GOULDING, Rui JIANG, Davey JONES, Xiaotang JU, Hongbin LIU, Yuelai LU, Lin MA, David POWLSON, Robert M. REES, Ute SKIBA, Pete SMITH, Roger SYLVESTER-BRADLEY, John WILLIAMS, Lianhai WU, Minggang XU, Wen XU, Fusuo ZHANG, Junling ZHANG, Jianbin ZHOU, Xuejun LIU. Progress on improving agricultural nitrogen use efficiency: UK-China virtual joint centers on nitrogen agronomy. Front. Agr. Sci. Eng., 2022, 9(3): 475‒489 https://doi.org/10.15302/J-FASE-2022459
Previous article Next article
1 INTRODUCTION
In recent years, China has been successful in feeding 20% of the world’s population with only 9% of the global cropland area[1]. Grain yield has increased substantially, with 57% of this increase being attributed to the use of synthetic nitrogen fertilizer[2]; China now consumes around 22% of the global synthetic N fertilizer (International Fertilizer Association statistics for year 2019). However, the low efficiency of N use through overuse and/or misuse of fertilizer has contributed to serious environmental pollution[3–5], increasingly threatening the sustainability of food production[6]. The challenge (and not just for China) is therefore how to maintain or increase food production while reducing environmental impacts of reactive N (Nr)[7,8]. The large increase in fertilizer N consumption in China in recent decades has resulted in a considerable excess over that required to meet food demand, indicating a poor average N use efficiency (NUE), and considerably above what could be regarded as the critical threshold in terms of environmental Nr loads (to air and to water) (Fig.1). After 2015, the estimated input required to meet the demands for increased food production surpasses the critical threshold for environmental impact, suggesting that improvement in NUE will be needed if future demand for food is to be met without exceeding critical Nr loads. Integrated analysis (unpublished data) has shown that opportunities exist to improve N management to reconcile these tensions between food demand and environmental impact in China, but this will require thorough reform of nutrient management across the entire food-chain system. This will not be simple because of the vast number of smallholder farms, inadequate knowledge or equipment, and a weak agronomic extension service[9].
Fig.1 Fertilizer N use (actual input) in China from 1950 compared with the estimated fertilizer N input required to meet food demand assuming an achievable nitrogen use efficiency throughout the food chain of 0.16 (required input) and the critical input in terms of environmental N loading whereby the N surplus meets environmental protection targets for air and water (unpublished data) |
Agriculture in the UK, by contrast has had static production in recent years but has built a strong knowledge-base and infrastructure for pollution control (although yet to fully realize and implement potential solutions). In this context, two virtual joint centers (VJC) on N agronomy were established between the UK and China in 2016, CINAg (Centre for Improved Nitrogen Agronomy) and N-CIRCLE, with the aim to deliver realistic short-to-medium term solutions for both countries in improving agricultural NUE to meet this double objective of maintaining/increasing food supply while minimizing environmental impacts of excessive Nr. This might be summarized as intensified crop productivity supported by near-closed-loop N cycling. Collaborative activities of the VJC included four key areas on which this review will focus: (1) improving fertilizer NUE; (2) management and use of livestock manures to reduce environmental impact and improve N use; (3) a better understanding of soil health and its role in improved cropland NUE; and (4) recommendations for policy development and knowledge exchange activities to realize improvements. This paper provides an overview of the research of the VJC in these four key areas and also comments on lessons learned through this large collaborative program.
2 IMPROVING FERTILIZER NITROGEN USE EFFICIENCY
Various management strategies exist, and were explored within the VJC, to improve the efficiency of use of applied inorganic N fertilizer. These included better matching of application rates to crop requirements, improved crop cultivars, the use of enhanced efficiency fertilizers, alternative system design and mulching in low rainfall areas. Over-application of N inputs to Chinese cropping systems is widely recognized as an important contributor to environmental problems[10]. This was highlighted in research by the VJCs that showed that there was an exponential increase in N2O emissions when fertilizer N applications exceeded optimum rates leading to a potential underestimate of cropland emissions[11]. Robust indicators of N management are required to assess the efficacy of management strategies, whether through observation or simulation modeling. While in itself not a means to improving NUE, the accurate quantification of gaseous Nr fluxes associated with different management activities and technical innovations is an important part of their assessment and development of gas flux measurement and analysis methodology was part of the VJC program.
2.1 Indicators of N management
Development of indicators with appropriate guidance benchmark values is an important step toward the improvement of N use in cropping systems. Two particularly useful indicators which can be used across a range of spatial and temporal scales are NUE and N surplus. Nitrogen use efficiency has several definitions, generally aimed at assessing the efficiency of applied fertilizer N, according to, for example, agronomic or physiologic efficiency, total N recovery or partial factor productivity[12]. The NUE balance provides an alternative indicator enabling a more comprehensive assessment of agronomic and environmental performance by taking account all the N inputs including inorganic and organic fertilizers, atmospheric deposition, and biological N fixation, provided that any soil N stock changes are small relative to the total N inputs[13].
The N surplus, the balance between total N inputs and N in harvested outputs[14], provides another indicator which is useful to use alongside NUE. Benchmark values for N surplus based on recommended optimum N management for given cropping systems can be developed for guidance, but there are few studies providing this information for cropping systems in China. Therefore, within the joint programs we developed benchmark N surplus values under optimal N management practice for the major cropping systems in China, based on results of on-farm field experiments (> 4500)[14]. Double cropping systems were associated with an N surplus of almost twice that of single cropping systems, with respective average values of 160 (range 110–190) and 73 (range 40–100) kg·ha−1·yr−1 N, with respective fertilizer N inputs in the range 294–381 and 127–184 kg·ha−1·yr−1 N and respective NUE values in the range 52%–70%, and 57%–80%. These N surplus benchmarks provide targets for improved N management practices in the cropping systems of China, and may be further refined as more innovative practices are introduced.
2.2 Nitrogen application rates and improved crop cultivars
The classic N response curve implies that NUE will decrease with increasing N application rate. It is important, therefore, that NUE is also set in the context of a given desirable minimum productivity and maximum N surplus[15]. The economic optimum N application rate will vary, depending on the relative prices of the commodity (e.g., wheat grain) and N fertilizer. An analysis of wheat yield gains associated with breeding programs in the UK since the 1980s has shown that the optimum N application rate has also increased, therefore there has been little or no improvement in NUE[16]. In contrast, there have been gains in NUE for spring barley, and the underlying reasons for the differences between crops may give useful pointers to the development of new cultivars and also means of assessment of cultivars[16]. Building on this as part of the VJC, Swarbreck et al.[17] proposed a new concept, with a focus on N responsiveness as a more appropriate trait to select for cultivars with a lower N requirement; cultivars showing a steeper N response curve reaching an economic N optimum at lower application rates. Finally, the sensitivity in the economic optimum N application rate to N fertilizer prices means that recommended rate changes in response to increasing fertilizer costs will be associated with an increase in NUE, although some decrease in yield[14,18]. At such higher input costs, the economic optimum sits on a steeper part of the N response curve, and the precision with which the target application rate can be achieved becomes more important.
2.3 Enhanced efficiency fertilizers
While not necessarily a panacea for resolving the N problem[19], the use of N fertilizers containing urease and/or nitrification inhibitors can significantly reduce Nr losses to the environment and improve crop yields[20], or maintain yields at a lower N application rate. Field experiments in spring maize grown in north-western China showed that optimum N fertilization with irrigation and a nitrification inhibitor could reduce cumulative N2O losses by 66% and increased NUE by 70% when compared with conventional farming practices, with the effect of the nitrification inhibitor alone reducing N2O by 34% and increasing NUE by 14% when compared to the optimized N application and irrigation rate treatment[21]. In the same study, the use of optimized N application and irrigation rate combined with slow-release N fertilizers reduced cumulative N2O losses by 59% and increased NUE by 66%, with the slow-release fertilizer giving a 14% reduction in N2O emission and a 9% increase in NUE compared to the optimized N application and irrigation rate treatment. Understanding factors influencing their efficacy is important to optimizing their use; in a meta-analysis, Sha et al.[22] identified soil pH, organic matter content, inhibitor compound and N application rate as the most important explanatory variables for the impact of inhibitors on fertilizer N recovery. Sha et al.[23] showed through laboratory and field experimentation that a urease inhibitor amendment had greater potential than a nitrification inhibitor amendment to reduce Nr losses from urea under the climatic and soil conditions of the North China Plain. Use of inhibitors within fertilizer blends or compound formulations, and storage conditions, can also influence their effectiveness in the field[24]. Carswell et al.[25] reported significant reductions in ammonia emission when a urease inhibitor was included with urea, relative to urea alone, at two grassland sites with no impact on nitrous oxide emission (also reported by Cowan et al.[26]) or yield. Carswell et al.[25] also discussed the importance of including wider environmental societal cost-benefit analysis into the choice of fertilizer type, demonstrating that the wider costs of urea fertilizer to society were 1.7–3.3 times more expensive than using urea with a urease inhibitor.
Inhibitors may also influence gross N transformation rates in the soil. Zhu et al.[27], using N15 as a tracer to assess the effect of the nitrification inhibitor, 3,4-dimethylpyrazole phosphate, reported significant reductions in N2O emissions from a UK and Chinese soil (by 22% and 9%, respectively), associated with lower gross N mineralization rates and gross autotrophic nitrification rates in both soils. The lower reduction effect observed for the UK soil was linked to the higher clay and SOC content of that soil, with a greater adsorptive capacity. Fu et al.[28] examined the effects of enhanced efficiency fertilizers on the soil microbiome, specifically nitrifier abundance and activity in soil, finding only a transient decline in the abundance of bacterial ammonia monooxygenase following application of the nitrification inhibitor dicyandiamide. However, the study of Fu et al.[28] was limited to a single year, at one site, consequently the impact of long-term enhanced efficiency fertilizer use on the soil microbiome and potentially the wider ecology of the surrounding environment is currently unknown.
Urease and nitrification inhibitors can also be combined with organic wastes, particularly low dry matter materials such as slurries and digestates (e.g., Vallejo et al.[29]). The nitrification inhibitor, 3,4-dimethylpyrazole phosphate, was incorporated with digestate from the anaerobic digestion of food waste and applied to a winter wheat crop at two UK sites, giving up to a 50% reduction in N2O emissions relative to digestate without the inhibitor[30]. However, the inclusion of the nitrification inhibitor did not result in any significant grain yield benefit, so the inclusion of the nitrification inhibitor to reduce N2O emissions represented a real net economic cost for the farmer.
2.4 Plastic film mulching
Plastic film mulching is now widely used in NW China to increase crop yields in arid and semiarid areas. A meta-analysis by Ma et al.[31] showed that plastic film mulching increased grain production by an average of 43.1% (ranging from 19.8% to 79.4% for maize, wheat and potato), compared with traditional non-mulched cultivation, with the main reasons given as increases in water use efficiency and NUE. They suggested that benefits may be influenced by crop type, rainfall and N application rate, and that full account needs to be taken of environmental impacts, including on N losses, in assessing the sustainability of the practice. Crop zoning and appropriate N application rates are recommended for plastic film mulching systems to achieve the largest yield and NUE. Under a ridge mulch system, inorganic N was shown to increase substantially and accumulate in the topsoil layer under the mulched ridge, compared with flat field non-mulched cultivation[32], and a high N application rate posed a risk of nitrate leaching, suggesting that N application rates could be reduced for the ridge mulch system, sustaining crop yield while reducing N losses to the environment. Using N15-labeled urea, Guo et al.[33] showed that plant uptake of the applied urea was 72.5% greater for the ridge mulched system, and hence losses to the environment much lower, with the ridge representing the main source for plant N uptake and the furrows the main source for N losses (mainly by ammonia volatilization). They concluded that NUE could be maximized by appropriately located N application into the ridges, from where plant uptake is greatest, and/or through the use of N fertilizer types other than urea (e.g., KNO3) that are associated with much lower losses through ammonia volatilization. Zhang[34] recently demonstrated that practices including split applications of N fertilizer, fertilizer N application just to the ridges, N application based on the topsoil (0−60 cm soil layer) nitrate content before sowing, and use of slow-release fertilizer instead of urea, could enhance NUE and crop yield and reduce N losses under plastic film mulching systems.
The use of plastic film mulching for cropping in arid and semiarid regions combined with appropriate fertilizer N application management can therefore greatly enhance crop yield and fertilizer NUE compared with non-mulched systems. Further work to understand the soil N transformations, uptake and losses for different cropping systems, soils and regions, and any impacts of using biodegradable plastic mulch compared to the conventional non-biodegradable type, will enable the development of clear farmer guidance.
2.5 Cropping system design
The individual management interventions described above can contribute to important improvements in NUE and reduced losses. However, it is important to consider management interventions within the framework of the whole cropping system to fully capture the trade-offs associated with different components of the N cycle. A study of cropping systems on the North China Plain showed that improved system design involving alternative crop rotations could improve fertilizer NUE by between 27% and 44% while reducing losses to groundwater[35]. The inclusion of soybean within these crop rotations decreased reliance on external fertilizer N inputs and therefore resulted in a corresponding decrease in the carbon footprint of the system. Legume-based rotations have also been shown to improve the NUE of rice-based systems in the south of China. A comparison of rotations with and without a legume green manure (milk vetch) showed that rice yields could be maintained in the presence of the green manure even when N fertilizer inputs were reduced by 20% as a result of the biological N input from the legume[36,37]. The incorporation of legume green manures also altered the structure of soil microbial communities[38], with recent evidence demonstrating a reduction in ammonia oxidizing archaea in soils receiving legume green manures[39]. This in turn reduced nitrification rates which may result in lower N losses from nitrification, denitrification and leaching. The SPACSYS model was used to investigate the responses to different management practices in seed yield, biological N fixation, seed N content and soil N budgets for soybean cropping in north-east China[40]. Scenario testing showed that irrigation at the reproductive stage improved seed yield in drier years with an increase of 12%–33% compared with that on rainfed. Biological N fixation was suppressed by fertilizer N application and drought stress, with decreases of 6%–33%, and 8%–34%, respectively. From this modeling study, it was recommended that applying fertilizer at 25–30 and 15–20 kg·ha−1 N before sowing is advised in drier and wetter years, respectively, in order to attain higher seed yield, and an application rate of 55–60 and 45–50 kg·ha−1 N is recommended for drier and wetter years, respectively, to achieve a higher seed N content.
Cui et al.[41] modeled how the uptake of improved management practices (integrated soil-crop management system) across smallholder agriculture in China, based on results of field trials across the major agroecological zones, had the potential to increase average crop (maize, rice and wheat) yield by about 11% with a reduction in N fertilizer use of 15%–18%. Modeling was also applied to UK grazing systems, using data from the North Wyke Farm Platform[42] to examine the N and C budgets and NUE of grassland swards at different stages of establishment[43]. The results showed that the NUE of the livestock production system was significantly greater for a mixed sward of a high-sugar grass with white clover (by 32%), especially in the year following reseeding (by 42%) compared with the other swards on the platform (permanent pasture or high-sugar grass alone).
2.6 Development of gas flux measurement and analysis methodology
Quantifying losses of Nr to gaseous forms of pollution such as N2O and NH3 can be difficult in small scale experimentation, largely due to the spatial and temporal influence of environmental and management factors. Due to its highly adsorptive nature, flux of NH3 is particularly difficult to measure in plot scale experimentation. As well as wind tunnel studies (e.g., Carswell et al.[25]), flux interpretation by dispersion and exchange over short range inverse dispersion model[44] was further developed and successfully deployed in fertilizer field trials for the first time[45], demonstrating that micrometeorological methods can be used to measure NH3 fluxes within a plot grid (16 plots), with each treatment plot covering an area of 20 m by 20 m.
Chamber methods are a commonly used and relatively simple technique for measurement of N2O flux; however, interpolation of results is difficult due to the unpredictable spatial and temporal nature of N2O emissions at the field scale. The first long-term eddy covariance measurements of N2O fluxes in the UK[46] highlight this is not just an issue with chamber methodology, and that even micrometeorological methods that can interpolate over time and space at the field scale suffer difficulties when gap-filling is attempted. This is partly due to exponentially large spikes in emissions following fertilizer events, and partly due to inconsistency in observed emissions even under similar management and meteorological conditions. Improvements in the analysis of chamber measurement data can be made by application of statistical methods which better address the log-normal characteristics of N2O flux measurements. The use of Bayesian methods to interpolate chamber data are one way in which to improve the estimate of cumulative fluxes and to better constrain uncertainties[26,47]. Using Bayesian statistical methods in future field studies is key to improving understanding of uncertainties and suitability of methodology to establish statistical significance between treatment types.
3 MANAGEMENT AND USE OF LIVESTOCK MANURE
There is great potential for a reduction in the use of inorganic fertilizers and in improving soil organic matter through the efficient and effective use of livestock manure. In the UK, where the majority of livestock manure is applied to the land, there is still scope for reducing N losses to the environment and improving nutrient use. In China, with a rapidly growing livestock sector[48], there is huge opportunity to substitute inorganic fertilizers with livestock manure, if the current barriers to use can be overcome and sustainable management systems developed. Nitrogen losses from the manure management chain in China were estimated to account for 78% of the N excreted by livestock in 2010, primarily through ammonia emissions and direct discharge to water bodies or landfill[49]. However, there is great scope for mitigation of these N losses through the implementation of appropriate management practices and technologies[48,50,51]. One issue, not specific to China but common to many regions globally, is the spatial polarization of intensive livestock production and cropping, resulting in an accumulation of nutrients in the livestock areas through the importation of feed and lack of export of manure back to the cropping regions. Potential solutions to this problem include the processing of livestock manures to develop fertilizers which can be economically transported to and used in the cropping regions, and/or policies to encourage greater co-location of livestock and crop production.
3.1 Manure processing
In China, it is common to use manure separation as a processing stage, particularly on the increasing number of larger livestock farms[48], resulting in a (semi-)solid fraction and a liquid fraction. The solid fraction is typically then composted for use as a soil amendment, particularly for vegetable cropping. There are large N losses associated with composting, primarily as ammonia, and also concerns regarding residual heavy metal content of the composted product, creating barriers to its use. The development of treatment processes to improve the sustainability of composting and acceptability of the product was an important part of the VJC. Enclosed reactor composting systems were shown to be as economical as windrow composting systems, when used at scale, and associated with a reduction in nitrogen losses of 34%[52]. Including acidification or the use of additives with the feedstock could further mitigate emissions[53,54]. Furthermore, an innovative technique involving the application of an electric field to the composting material, binding the heavy metals into unavailable forms and reducing nitrous oxide emissions has been demonstrated at the pilot scale[55–57]. Acidification of waste streams to reduce ammonia emissions has also been gaining interest in the UK. Within the VJC, Sánchez-Rodríguez et al.[30] showed that acidification of digestate from the anaerobic digestion of food waste (a growing organic resource in the UK) substantially reduced N losses following application to land, by 95% for ammonia and by 50% for nitrous oxide.
3.2 Manure nutrient value
A significant barrier to effective manure nutrient use is a lack of knowledge of the nutrient content and plant availability of those nutrients for the different manure types associated with livestock systems in China[48]. The UK has a system of fertilizer recommendations for crops and grassland, including guidance on the nutrient content, availability and use of organic resources including livestock manure (RB209[58]), underpinned by models such as MANNER-NPK[59]. The development of such a system for China, with robust estimates of nutrient content and availability for different manure types based on a representative measurement program, would enable and encourage the integrated use of manure nutrients together with inorganic fertilizers, optimizing overall nutrient use with resultant decreases in nutrient leakages to the environment. Collation of existing experimental data toward this aim, with further work to fill existing knowledge gaps on nutrient content, availability and use across livestock and cropping systems, soils and climates in China, was highlighted by the VJC as a key research need. This should include promotion of and accounting for improved management practices through manure processing and/or application methods designed to minimize N losses to the environment and maximize use by the growing crop.
4 SOIL HEALTH
For agricultural soils, a healthy soil can be defined as a soil which can sustainably continue to deliver food production alongside other desirable ecosystem services[60]. The health of continuously cropped, heavily fertilized soils has declined considerably, including a loss of soil C, structure, increasing acidification and loss of biodiversity. Within the VJC, specific research objectives aimed to better understand the processes underlying the maintenance of good soil health and the links between soil health and NUE.
4.1 Metrics for soil health in agricultural soils
Metrics based on the biological, chemical and physical attributes of the soil can be useful proxies for representing the status of key soil processes, indicators of a healthy soil and, if measured across time, indicators of change and progress toward desirable target states. The VJC developed a framework, identifying metrics where there is good evidence of links to soil functioning in the context of improving NUE (Tab.1), which might be further developed either as key indicators or in terms of improving understanding of processes. Soil organic carbon (SOC) is commonly proposed as one of the most important metrics for soil health (as discussed below), but the importance of soil texture in this context, and the capacity for a soil to hold SOC is relevant in terms of developing indicators of soil health status. Prout et al.[61] suggested threshold values of a SOC/clay index as indicators of soil structural condition (classified from degraded to very good) for England and Wales across different land use types. Such an indicator, based on readily measurable soil properties, could also be developed for China giving a simple method to assess and monitor soil status at national, regional and local scales.
Tab.1 Framework for development of potential metrics for indicators of soil functions relevant to NUE |
Function | Biological | Chemical | Physical | |||||||||||
N cycling processes | Readily oxidizable C | Biological community | Microbial biomass C, N & P | N quantity and type | Soil organic matter | pH | Available P | Hydraulic properties | Bulk density | Texture | Aggregate stability | |||
Yield | ||||||||||||||
Gaseous N losses | ||||||||||||||
N leaching | ||||||||||||||
Resilience | ||||||||||||||
Water retention | ||||||||||||||
C sequestration |
Note: Shaded cells are where good evidence exists of relationships between a soil function and a measurable parameter, suggesting these may be useful metrics to develop. |
4.2 Soil carbon
The focus of the VJC was N, however, the N and C cycles are closely integrated in agricultural systems, and soil C is a major contributor to soil health (e.g., Lal[62]). Although much has been claimed for opportunities to build or rebuild SOC in farmed soils, a realistic assessment shows that what is possible is more limited[63]. The long-term experiments at Rothamsted Research clearly show which management practices tend to result in a decrease in SOC, such as continuous cropping and which increase SOC, such as permanent grassland and regular, large inputs of manure and other bulky organic materials[64].
Soil organic carbon stocks in China’s croplands had been in decline until the mid-1980s due to a transition to farming with continuous cropping and low inputs of organic and inorganic fertilizers[65]. However, since the mid-1980s, SOC stocks have begun to increase regionally (e.g., Liao et al.[66]) and nationally, which in turn has led to improved productivity and yield stability[67]. Evidence of increases in cropland SOC stocks have come from long-term field experiments[68–70], direct measurement via repeat sampling surveys[71,72] and via agricultural statistics[73,74]. The increase has been the result of policies to improve soil management, for example through green manures[75] or changing irrigation regimes for rice[76], but largely as a result of increased productivity, leading to greater inputs of crop residue C to the soil[72,77], initially through increased root biomass but more latterly as a direct result of large-scale implementation of the crop residue return policy[65]. Despite this recent increase, there remains substantial potential for further soil C sequestration in China’s croplands[78]. However, despite the importance of C sequestration in reducing overall greenhouse gas emissions, Chinese croplands were found to be a net source of greenhouse gas emissions due to the large emissions associated with soil N2O and CH4 emissions plus upstream CO2 emissions arising from agronomic management. A study by Gao et al.[79] demonstrated that between 2000 and 2017, despite an SOC increase of 23.2 Tg·yr−1 Ceq, N2O and CH4 emissions plus embedded upstream emissions associated with agronomic management added 269.5 Tg·yr−1 Ceq to the atmosphere, demonstrating the importance of improved N management in sustainable agricultural production. Concurrently, SOC stocks have continued to decline in grasslands[80] as have soil inorganic carbon stocks[81] as result of significant soil acidification[82,83].
Many management options to improve NUE, such as reducing over-fertilization, better use of organic amendments, nitrification inhibitors, switching fertilizer type and conservation tillage, also improve SOC stocks[84]. However, some practices aimed at increasing soil carbon stocks, such as straw incorporation and increased additions of animal manure, can increase N2O emissions, leading to a greater climate impact[84]. Recently, studies have shown that biochar additions to the soil can increase soil C stocks, reduce N2O emissions and improve productivity[84], whereas others have shown that digestate from biogas production can increase SOC and enhance returned straw decomposition in a wheat-rice rotation in China[85].
4.3 Soil acidification
Soil acidification rates have increased in many parts of the world, including important cropping regions of China, accompanied by decreasing crop NUE[3,86] due mainly to increased and long-term use of acidifying N fertilizers (urea and ammonium-based) and their associated N transformations within soil. The direct effects of long-term N fertilizer use only contribute to soil acidification when the fertilizer N is nitrified and the ensuing nitrate is leached from the soil, leading to proton accumulation; if the crop takes up all of the applied N, no net acidification occurs[87]. Consequently, in agricultural systems with low NUE, the capacity for soil acidification is great under urea or ammonium-based fertilizers, although inherent soil pH, buffering capacity and climate (with rainfall as the driver of leaching) will influence the actual rate of soil acidification. Zhu et al.[88] estimated that under a business-as-usual scenario of N fertilizer inputs increasing by 1% annually, yields of wheat, rice and maize will decline by 30%, 30% and 15% over the period 2010–2050. Another major contributor to soil acidification is plant uptake and removal of base cations from the soil[3,89]. Base cations are not typically replaced through conventional N fertilizer application, consequently their removal has direct implications for soil acidification and for plant nutrient availability.
Liming has widely been used to mitigate soil acidification and maintain optimum soil pH levels for crop production[83,84]. Using their VSD + model, Xu et al.[89] simulated soil pH and base saturation changes for two long-term experiments at Rothamsted Research and suggested that base cation uptake was the dominant process in soil acidification, with N transformations having a lesser effect. Using the same model, Xu et al.[90] calculated that the paddy and upland agricultural systems in Qiyang, China, required c. 2.5 t·ha−1 of lime to raise soil pH to 6.5. Although liming is an effective way to ameliorate soil acidification, it may often incur significant economic and labor resource costs[3].
Manure and crop residue amendments may represent an alternative strategy to ameliorate acidic soils[91–93]. Immobilization of NO3− and NO2− by crop straw residues with a high C:N ratio is one mechanism whereby soil pH is increased. Cai et al.[91] showed that swine manure, soybean straw and maize straw all significantly increased soil pH and base cations, and decreased exchangeable acidity in a red soil (Ferralic Cambisol). Swine manure was particularly effective in reducing exchangeable Al concentration. Straw return alone may be insufficient to effectively mitigate soil acidity and combination with other amendments, such as lime or manure, together with reduced chemical fertilizer input, may provide a feasible solution to long-term soil acidity management in these red soils. Manure incorporation is an effective management strategy for nutrient supply, animal waste utilization, and mitigation of soil acidification in the low productivity red soils. Cai et al.[93] showed that with 40% of the total N requirement provided by continuous swine-manure application, soil acidification was prevented and maize yields enhanced. The manure was also a significant source of P and no additional P input is required for manure application providing ≥ 20% of N requirement, but additional K input would be required. Accumulation of P and some heavy metals presents a challenge to the long-term use of manure for acidity management in China, but an integrated approach where manure is used to supply part of the N requirement, combined with reducing source heavy metal in animal feed and providing inorganic fertilizer to meet the remaining nutrient requirement represents a promising strategy for managing soil acidity and nutrient supply.
4.4 Soil biodiversity
Soil biodiversity is an important component of a healthy, functioning agricultural soil, and is generally reduced by many current intensive agricultural practices including tillage and high levels of fertilizer and pesticide use and the predominance of genetically-uniform monoculture cropping systems[94]. Changes in the diversity of the soil fungal and bacterial communities in agricultural soils can significantly impact the soil multifunctionality[95]. Soil ecological engineering is a concept of managing soils specifically to enhance the ecosystem service delivery (healthy functioning agricultural soils in this context) as driven by the soil biodiversity[94]. This may be through broad-scale interventions to create the conditions favoring increased biodiversity, at the plant-level including practices such as polycultures with different rooting characteristics, cover cropping, intercropping and crop rotations, and at the soil-level including practices such as reduced or zero tillage, application of organic materials and mulching. It may also be through direct manipulation of soil communities to enhance the ecosystem delivery including interventions at the rhizosphere exploiting specific plant-microbial interactions, and direct soil inoculations with beneficial organisms. However, more understanding is required in terms of the specific effects of such interventions and their interactions with other factors including soil type, climate, fertilizer use and cropping systems, to enable more specific recommendations regarding agronomic practices.
5 POLICY DEVELOPMENT AND KNOWLEDGE EXCHANGE
The work of the VJC has had an important contribution toward the development of policies in China concerning sustainable agricultural production and environmental protection and the provision of evidence to underpin such policies.
China is committed to improving environmental quality while maintaining economic growth and food production from the agriculture and land use sector[96]. This balancing act underpins policy development in agriculture and the wider economy and nowhere is this more evident than in the recently developed climate change mitigation policy. China plans to reach net zero greenhouse gas emissions by 2060[97], but to achieve this there will need to be significant changes in agricultural production. China’s croplands are a major source of global anthropogenic N2O emissions[98], and improved N management will therefore be critical to achieving the national net zero policy target.
The overuse of N fertilizers has been recognized as an important factor contributing to inefficient nutrient recovery and environmental degradation by policymakers. This led to the introduction in China of the Nationwide Soil Testing and Formulation Fertilization Program in the early 2000s and recommendations for altered spatial distributions of crops[99]. These changes have resulted in a reduction in fertilizer N use and lower emissions of N2O. Thus, while China’s cropland N2O emissions increased by 11.2 Gg·yr−1 N between 1990 and 2003, emissions subsequently stabilized in response to policy interventions[99]. In 2015, the Chinese Ministry of Agriculture initiated the Action Plan for Zero Growth in Fertilizer Use by 2020, requiring a change in agricultural production from a high productivity, high input, and high pollution mode to a high productivity, high efficiency, and environmentally friendly mode. Increasing awareness of pollution issues connected with livestock manures, and the move to large, landless livestock production systems, prompted policies around the prevention and control of pollution from intensive animal farms and a requirement for manures to be recycled to land.
A recognition of the importance of system design in contributing to reduced N pollution was published by the Chinese Ministry of Agriculture in the Plan to Adjust Planting Structure (2016−2020), where it was recommended that maize cultivation should be reduced to allow increased production of soybean (Ministry of Agriculture and Rural Affairs of China 2016). The results of research carried out by the VJC also support government policies to subsidize the cultivation of green manures in place of winter wheat cultivation and the introduction of fallow periods in order to protect ground water supplies (Ministry of Agriculture and Rural Affairs of China 2019).
It is important also to recognize potential trade-offs as a consequence of specific actions. For example, relocation of swine in China from some areas in response to a policy of banning livestock production in certain regions (non-livestock production regions) to protect surface water quality increased the risk of groundwater and atmospheric pollution in other regions of China[100]. In other areas, current policies may not have gone far enough; Bai et al.[101] suggest that China’s Blue Sky Protection Campaign, which set targets for SO2, NOx and PM2.5 emissions should also include targets for ammonia, while also accounting for interactions between air quality and water pollution measures to assess risks of trade-offs. Nitrogen is obviously only one aspect of sustainable agriculture, but with a focus specifically on NUE and reducing losses of Nr to the environment, the VJC has also made a valuable contribution to the Agriculture Green Development program in China[102], a major focus of which is the reconstruction of crop-animal production systems and food production-consumption systems.
Policies will only deliver benefit if effectively enacted, and this requires mechanisms for effective knowledge exchange, and support and incentives where necessary, to bring about the implementation of improved practices. The structure of agriculture in China, with many regions dominated by smallholder farms, makes this challenging; however, the Science and Technology Backyard program has proved to be a successful model for direct engagement with smallholders at the village scale, bringing about improved practices through training and demonstration and having impact at scale[41,103]. The formation of village-scale cooperatives for fertilizer delivery and blending, for example, was observed during the VJC to be particularly successful in enabling smallholders to access the correct amounts and formulations of fertilizers, where previously they would have applied in excess (as standard bag sizes provide too much) with little regard to the balance of nutrients. This model might be successfully applied in other world regions, such as India, where smallholder farming dominates. However, it is also important to engage with larger operators and associated agricultural industry supply and support companies as agriculture structural development takes place in China, enabling improved practices to be built in at an early stage and more cost-effectively. One example of this is the engagement of industry in the development and scaling up of the intelligent reactor composting system[52], now being deployed directly on farms and at centralized processing centers.
6 LESSONS LEARNED FOR LARGE COLLABORATIVE PROJECTS
The development, writing and eventual success of the grants were facilitated by the already effective research relationships between UK and Chinese colleagues, built over many years via bidirectional international travel awards and other exchange visits. New collaborations take time to develop and become fully productive, and these prior activities provided a strong base from which the VJC could rapidly progress. This highlights the benefits to funders of planning for continuity of successful and productive engagements. Full benefits can best be realized with equal engagement and equivalent funding provision from both sides. The VJC addressed a very policy-relevant research area which ensured strong engagement from all partners. Continued engagement was supported through regular meetings (we convened an annual cross VJC workshop in addition to more frequent project-focused meetings), communications, staff exchanges and developing joint publications and other outputs. Research partners also benefited from the development of harmonized experimental and modeling protocols.
A missed opportunity was the lack of an aligned doctoral training program, jointly funded, with co-supervision from China and the UK. Although we did benefit from some student exchange (predominantly from China to UK), a defined and coordinated PhD program would probably have realized greater benefits. Additionally, greater use of common experiments, treatments and platforms from the outset of the program would have added benefit to the VJC and aided in greater synthesis of the results. Finally, it was evident that the natural science research conducted showed what is possible in terms of improving nitrogen agronomy with new and developing techniques, but inclusion of social science is also needed to investigate the social changes (e.g., farm size, management and farmer age) needed to make change happen on the ground.
7 CONCLUSIONS
The establishment of the VJC enabled effective collaboration across multiple institutions in China and the UK, building on a strong base of prior engagement, with the common aims of improving agricultural NUE while maintaining or increasing food production and reducing losses of Nr from agricultural production systems to the environment. The research conducted through the VJC has contributed to the identification and assessment of potential management practices and interventions toward these aims, including integrated nutrient management utilizing organic and mineral fertilizer resources, development of nutrient recommendation systems, use of enhanced efficiency fertilizers and manure processing technologies and an improved understanding of the key aspects of soil health. The research conducted highlighted the importance of developing a systems-level concept of N management to ensure improvements in overall N efficiency and the avoidance of unintended secondary effects through individual interventions. Within this context, management of fertilizer emissions and improved manure management at farm and regional scale appear to be particularly important targets for mitigation. The VJC have contributed to and influenced policy development and demonstrated the potential for improvements at farm and regional scale. The need for the development of innovative solutions, knowledge exchange and widescale implementation of improved practices continues, and the connection of the stakeholders, researchers, industry and policymakers, at scale, as in the VJC, is an effective mechanism to deliver this.